(written by: Willy Yanto Wijaya)
Abstract
In electricity generation plants, energy storage system is very important. Demand of electricity from consumers changes from time to time. For conventional power plants, the electricity generated at off-peak time can be stored to be used later at the peak time, thus enabling the constant operation of power plants and enhancing efficiency. The roles of these energy storage systems are even more obvious in the renewable energy power plants (such as wind and solar energy) where intermittence (fluctuation) of energy sources’ input is significant.
In this section, several types of grid energy storage will be discussed; highlighting their pros and cons. Focus will be put on three main types of grid energy storage: pumped-storage hydroelectricity, battery, and hydrogen energy.
Introduction
Energy storage technologies are essential parts of today’s civilization development. Substantially, energy can be stored in many forms: mechanical, thermal, chemical, nuclear, electrical, magnetic-field, and even in the form of matter (mass) itself. However, in this section, we shall only discuss about energy storage technologies for electricity grid infrastructure.
As we know, demand of electricity keeps changing from time to time. In the peak time, the demand of electricity might increase significantly; whereas demand might decrease so much at the off-peak time (for example at midnight). Therefore, the power plant companies have to adjust the amount of electricity generated to meet this changing demand. At the same time, we know that some types of power plant require constant operation in order to have a good efficiency as well as longer life-time. Thus, excess electricity generated from these power plants can be stored in energy storage systems first, which might be used later when the demand of electricity becomes high.
In addition, besides the changing demand, some types of renewable energy sources also generate electricity intermittently, such as wind and solar energy. We also know that electricity is the flowing of charge (electron) and therefore can’t be stored in the form of electricity itself (except in very few amounts as static charges in capacitor). Therefore, we have to convert this electricity and store it into other forms of energy.
Three main types of energy storage technologies will be introduced. They are: pumped-storage hydroelectricity, battery storage, as well as hydrogen energy.
Pumped-storage Hydroelectricity
The schematic of pumped-storage hydroelectricity is illustrated in Fig.1. The basic idea of this pumped-storage hydroelectricity is that the excess electricity generated (for example at midnight when the price of electricity is cheap) will be used to pump up the water in the lower reservoir (river) to the higher reservoir (dam). This pumped-up water will then be used later to flow down rotating the turbine and generate the electricity at the peak-time (when the price of electricity is expensive).

Fig.1. Schematic of Pumped-storage Hydroelectricity
Besides in hydro-power plants, there is also possibility of applying this energy storage technology in the integrated thermal – hydro plants, such as shown by Fig.2.
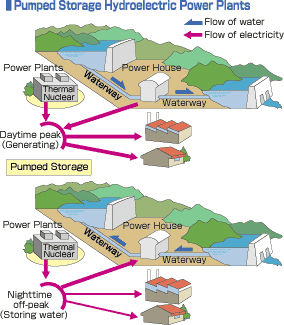
Fig.2. Applying hydro-storage in integrated thermal-hydro power plants
Here, as we can see in Fig.2, at the daytime peak, the stored hydro-energy will be used to be combined with the electricity generated by thermal/ nuclear power plants to meet the demand of electricity from consumers. Meanwhile, at the night-time off-peak, the electricity generated by these thermal/ nuclear plants will be used to pump up the water to the higher reservoirs.
Fig.3 shows Aldeadávila Dam, one example of hydro-power plant that utilizes the technologies of pumped-storage hydroelectricity. This dam is a type of gravity-arch dam on the Duero River which forms the border between Spain and Portugal. The dam is 56 miles west northwest of Salamanca, Spain.

Fig.3. Aldeadávila Dam, a type of gravity-arch dam utilizing pumped-storage hydroelectricity.
Well, so what are the pros and cons of this type of energy storage? Several advantages of pumped-storage hydroelectricity:
- Can recover 75% of the energy consumed
- Currently most cost effective form of mass power storage
- High dispatchability (15 sec), effective at soaking up demand variability
- Total 90 GW pumped storage (equal 3% instantaneous global generation capacity)
However, this type of grid energy storage also possesses drawbacks as follow:
- Considerable initial capital expenditure
- Require suitable geographical terrains
Battery Storage
Since pumped-storage hydroelectricity will best fit if suitable geographical terrains exist, then whenever this geographical condition can’t be feasibly afforded, battery storage technologies might then become another possible option. Battery can be classified into two main types: primary battery and secondary battery. Primary battery is the type of batteries that cannot be recharged, whereas secondary battery is rechargeable. Since we are discussing about grid electricity storage, thus only secondary battery might be used for the purpose of electricity storage.
Secondary battery can be divided further into two categories, i.e. the closed type and the flow type. In the closed type battery, the electrode and the electrolyte are in still condition. One example of this closed-type secondary battery that is currently being used as grid energy storage in power plants is the sodium-sulfur battery, which is schematically shown by Fig.4.
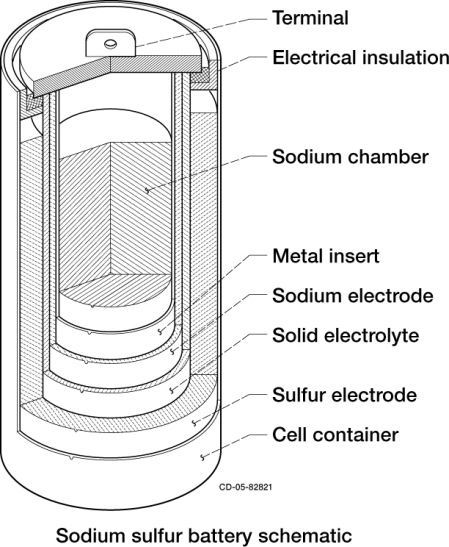
Fig.4. Schematic of sodium-sulfur battery
When sodium (Na) gives off an electron, the Na+ ion migrates to the sulfur container. The electron drives an electric current through the molten sodium to the contact, through the electrical load and back to the sulfur container. Here, another electron reacts with sulfur to form Sn2−, sodium polysulfide. The discharge process can be represented as follows:
2 Na + 4 S → Na2S4 , where Ecell ~ 2 V.
As the cell discharges, the sodium level drops. During the charging phase the reverse process takes place. Currently, this battery can last for about 2500-4500 cycles and possess efficiency about 87%.
Meanwhile in the flow-type secondary battery, the electrolyte flow through electrolyte tanks/ pipes, as shown in Fig.5. One example of this battery type is vanadium redox battery. A vanadium redox battery consists of an assembly of power cells in which the two electrolytes are separated by a proton/ ion exchange membrane. Both electrolytes are vanadium based, the electrolyte in the positive half-cells contains VO2+ and VO2+ ions, the electrolyte in the negative half-cells, V3+ and V2+ ions. When the vanadium battery is charged, the VO2+ ions in the positive half-cell are converted to VO2+ ions when electrons are removed from the positive terminal of the battery. Similarly in the negative half-cell, electrons are introduced converting the V3+ ions into V2+. During discharge this process is reversed and results in a typical open-circuit voltage of 1.41 V at 25 °C.
The efficiency of this vanadium redox battery is about 65-75%. The energy density of the electrolyte is about 10-20 Wh/ kg.

Fig.5. Schematic of a typical flow battery
In general, thus, we can list several advantages of battery energy storage as follow:
- Very quick response time (can be in order of sec)
- Efficiency as high as 90%
- No emission
- Many off-the-grid domestic systems can rely on battery storage.
Meanwhile, battery technologies still have several drawbacks as follow:
- Generally expensive, high maintenance, limited life-span
- Low energy density (proportional to the size)
- Chemical waste recycling and handling.
Hydrogen Energy
Another exciting type of energy storage is hydrogen energy. Based on thermodynamics, 1 kg of H2 (500 mol) has chemical energy content equal 143 MJ (40 kWh). This is very high compared with the (for example) electrolyte of vanadium redox battery which has the energy density only about 10-20 Wh/ kg. However, in term of power density (response time – amount of electricity generated in very short period), generally battery system is better, as shown by Ragone chart (Fig.6).
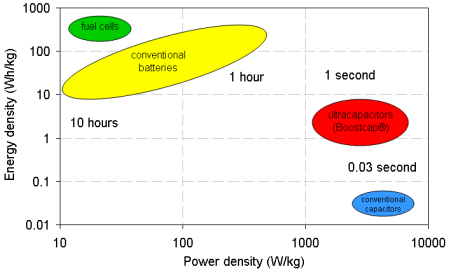
Fig.6. Ragone-chart of several types of energy storage systems.
Hydrogen can be produced in many ways, such as reforming reactions of hydro-carbon as well as through many types of water-electrolysis and thermal dissociation. A typical example of electrolysis reactions of water is shown as follow:
Anode (oxidation): 2H2O (l) -> O2 (g) + 4H+ (aq) + 4e–, Eox = -1.23 V
Cathode (reduction): 2H+ (aq) + 2e– -> H2 (g), Ered = 0.00 V
Total reaction: 2H2O (l) -> 2H2 (g) + O2 (g), E = -1.23 V
where the chemical kinetics formula can be written as:
G = – n F E.
Since the value of E of the total reaction is negative, F (total charge of 1 mol electron) about 96485 Coulomb/mol, and n is the moles of electron, therefore the Gibbs energy (G) will have positive value, which mean that this electrolysis reaction require input energy. The efficiency of electrolysis of water currently is about 50-80%.
Hydrogen fuel produced can then be later utilized in the Fuel Cell system. A schematic of typical PEFC (polymer electrolyte fuel cell) is shown by Fig.7.

Fig.7. Schematic on how PEFC works
Hydrogen energy has several advantages as follow:
- Clean and friendly for environment
- Many ways of production
- Portability and high energy density
- Development of technologies utilizing H2 such as fuel cell.
Meanwhile, it also still possesses several drawbacks i.e. lacking of:
- Hydrogen Storage Technology
- Development of Hydrogen Infrastructure
- Efficiency enhancement of Hydrogen Production
- Currently still expensive.
However, if the technology for this hydrogen storage and infrastructure could be developed progressively, hydrogen energy will become a very potential candidate of future fuel and promising form of energy storage.
Conclusion
The development of energy storage technologies is very crucial for the electricity generation and grid infrastructure. Each of these storage technologies possesses its pros and cons. Therefore, it is necessary to combine these technologies and utilize them for applications which suit them in the best way, respectively.
References
[1] D.A.J. Rand, Hydrogen Energy – Challenges and Prospects, RSC Publishing, 2008.
[2] Frano Barbir, PEM Fuel Cell: Theory and Practice, Academic Press, 2005.
[3] George Olah, Beyond Oil and Gas: The Methanol Economy, Wiley-VCH, 2006.
[4] Norio Sato, Chemical Energy and Exergy, Elsevier Science, 2004.
[5] Saiful Hasmady, In situ Visualization of PEFC Inner Reaction and Transport Phenomena, EPL-Tokyo Tech 2007.
[6] W.Y. Wijaya, K. Okazaki, K. Fushinobu, A. Waris, Feasibility Study of Integrating Absorption Heat Pump into Methanol Steam Reforming Process for Hydrogen Production, Proceeding of the 2nd Asian Physics Symposium, 2007.
[7] http://www.tepco.co.jp/en/challenge/energy/hydro/power-g-e.html
[8] http://www.window.state.tx.us/specialrpt/energy
[9] http://web.hulteen.com/eric/portugal_2007.html
[10] http://www.mpoweruk.com/flow.htm